In this article, I briefly describe how CRISPR technology inactivates a gene and observes the resulting changes, helping scientists understand the gene’s normal function.
Introduction of the CRISPR technology
Gene mutation is necessary to understand the function of a gene. Researchers can then examine the effect of genomic alteration on cell growth or function. Introduction of a precise nuclease into a cell cuts the gene of interest at a functionally critical site. It is a common technique that generates a double-strand break. Cellular systems generally repair such breaks in eukaryotes. This promotes non-homologous end joining. During the repair, nucleotides are often added or deleted, inactivating the gene. However, the process involves imprecision. In bacteria, the double-strand breaks are usually repaired more accurately by homologous recombination. However, this process is quite expensive, which led to the introduction of the CRISPR technology in 2011. “CRISPR” stands for clustered, regularly interspaced short palindromic repeats.
CRISPR-Cas System: Bacterial defense mechanism against viruses
The CRISPR-Cas system is a specialized immune strategy adapted by bacteria to protect themselves from viral infections, particularly those caused by bacteriophages. In this system, the Cas protein functions as a nuclease, an enzyme that cuts DNA. The bacterial genome contains CRISPR sequences interspersed with segments of viral DNA, known as spacer sequences, which are remnants from past infections. These stored viral segments allow the bacterium to recognize and respond to future attacks by the same virus. When a familiar bacteriophage invades again, the CRISPR sequences guide the Cas protein to the matching viral DNA, enabling it to cut and neutralize the threat efficiently.
The CRISPR sequences are first transcribed into RNA, and the viral spacer regions are then processed into smaller units known as guide RNAs (gRNAs), which also contain parts of the nearby repeat sequences. These gRNAs associate with one or more Cas proteins to form an active complex. In certain types of CRISPR systems, the gRNA also pairs with an additional RNA molecule called the trans-activating CRISPR RNA, or tracrRNA, to facilitate the targeting process. The resulting complex binds specifically to the invading bacteriophage DNA. The complex is associated with Cas proteins having nuclease activities, which aid in cleaving and destroying the invading bacteriophage DNA.
The CRISPR/Cas9 system
A simple CRISPR/Cas system in Streptococcus pyogenes requires only a single Cas protein, Cas9, to cleave DNA. Jennifer Doudna and Emmanuelle Charpentier produced a streamlined CRISPR/Cas9 system. This consists of a single protein, Cas9, and one associated RNA. The RNA consists of gRNA and tracrRNA fused into a single guide RNA (sgRNA). The guide sequence can be altered to target almost any genomic sequence (Figure 1). Cas9 has two discrete nuclease domains. The two domains cleave different DNA strands. One domain cleaves the paired DNA strand with the sgRNA, and the other cleaves the opposite strand. One domain is inactivated to create an enzyme that cleaves only one strand, thus forming a nick (Figure 1). The sgRNA serves two main functions: it pairs with the target DNA sequence and simultaneously activates the nuclease domains to enable cleavage.
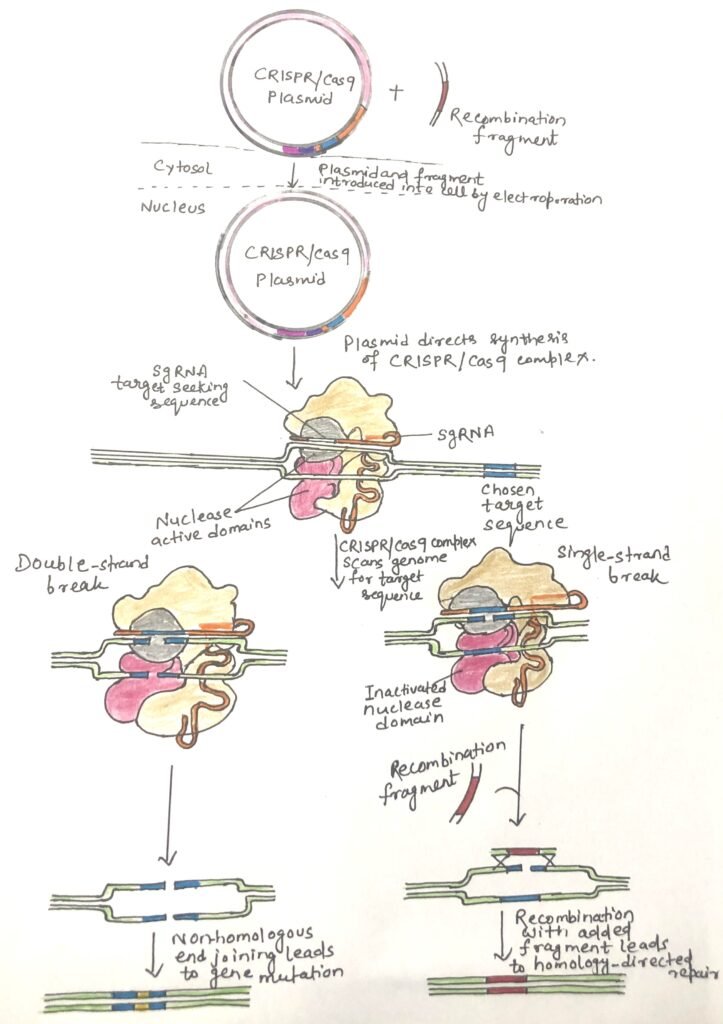
Enhancing gene editing efficiency with CRISPR/Cas9 and recombination strategies
Plasmids expressing the desired protein and RNA components of CRISPR/Cas9 can be delivered into cells via electroporation. In many organisms, this method leads to targeted gene inactivation in approximately 10% to 50% of the treated cells. When precise genomic alterations are needed rather than mere gene knockout, a donor DNA fragment containing the desired mutation and spanning the CRISPR target site can be co-introduced. This allows for site-specific recombination, although the efficiency of this process is typically low. However, introducing a single-strand nick instead of a double-strand break at the target site can modestly enhance recombination efficiency.
Innovative applications of CRISPR/Cas9 are emerging at a fast pace. While widespread therapeutic use is still years from realization, ongoing advancements are paving the path toward future treatments for genetic disorders, HIV, and a wide range of other human health conditions.
Conclusion
Gene mutation helps researchers understand gene function by observing the effects of genomic changes on cell growth or activity. Introducing a precise nuclease creates a double-strand break at a key gene site. In eukaryotes, this is typically repaired by non-homologous end joining, often causing insertions or deletions that inactivate the gene, though the process is imprecise. In contrast, bacteria use the more accurate but costly homologous recombination. To overcome these limitations, CRISPR (Clustered Regularly Interspaced Short Palindromic Repeats) technology was introduced in 2011.
The CRISPR-Cas system is a bacterial immune mechanism against viral infections, especially bacteriophages. It uses Cas proteins, which act as nucleases, to cut viral DNA. Bacteria store viral DNA fragments (spacers) in CRISPR sequences, enabling recognition of future infections. Upon reinfection, CRISPR sequences are transcribed into guide RNAs (gRNAs), which pair with Cas proteins to form a complex that targets and cleaves matching viral DNA. In some systems, gRNAs also pair with tracrRNA to enhance targeting.
Jennifer Doudna and Emmanuelle Charpentier produced a streamlined CRISPR/Cas9 system. This consists of a single protein, Cas9, and one associated RNA. The RNA consists of gRNA and tracrRNA fused into a single guide RNA (sgRNA). The guide sequence can be altered to target almost any genomic sequence.
Plasmids expressing the desired protein and RNA components, CRISPR/Cas9 components—both protein and RNA—can be delivered into cells via electroporation. In many organisms, this method leads to targeted gene inactivation in approximately 10% to 50% of the treated cells.
You may also like:
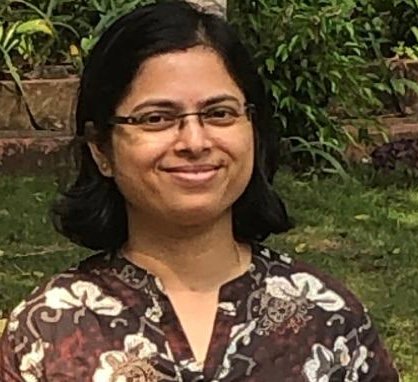
I, Swagatika Sahu (author of this website), have done my master’s in Biotechnology. I have around fourteen years of experience in writing and believe that writing is a great way to share knowledge. I hope the articles on the website will help users in enhancing their intellect in Biotechnology.