In this article, I briefly describe the two important globin proteins, hemoglobin and myoglobin, and their functions.
The globin proteins
The globin family of proteins having similar primary and tertiary structures is found in all classes of eukaryotes and some bacteria. The main function of globins is to store and transport oxygen. In mammals, there is the presence of four kinds of globins.
Myoglobin
It is found to abound particularly in the muscles of diving marine mammals, such as whales and seals. Myoglobin is a single polypeptide chain consisting of 153 amino acid residues. One molecule of heme occupies a space within the globin.
Myoglobin contains eight α-helical segments connected by bends and contains 78% of amino acid residues found in the protein. The eight helical segments are numbered from A to H. An amino acid is marked by its position in the sequence of amino acids or by its location in the sequence of a specific α-helical segment.
Hemoglobin
Hemoglobin is a tetrameric protein with four heme prosthetic groups, associated individually with each polypeptide chain. It is a roughly spherical protein with a diameter of around 5.5 nm.
Adult hemoglobin contains two alpha chains and two beta chains. Hemoglobin subunits are structurally similar to myoglobin, though the amino acid sequences of the three polypeptides are identical at only 27 positions. All three polypeptides belong to the globin family of proteins.
The binding of a ligand to a protein
The ligand-protein interaction is severely affected by protein structure and is accompanied by conformational changes. When heme is a component of myoglobin, the specificity with which it binds with its ligand is altered.
With free heme molecules, carbon monoxide binds more than 20,000 times better than oxygen, whereas, within myoglobin, the binding of CO and heme is only 40 times better than oxygen.
The more compact binding of carbon monoxide with free heme molecules shows the differences in the way the orbital structures of CO and O2 interact with Fe2+. When CO and O2 bind with free heme molecules, the orbital structures are related to different binding geometries.
When heme is a component of Myoglobin
However, in a globin molecule, as in myoglobin, when the heme is bound to a globin, the globin structure mediates the differences in relative affinity of CO and O2 for heme.
In myoglobin, when heme is attached, the affinity of Fe and O2 increases by the presence of distal His (His64, or His E7 in myoglobin). In a Fe-O2 complex, a partial negative charge is distributed across the oxygen atoms because of the partial oxidation of the interacting iron atom.
The Fe-CO is quite less polar than the Fe-O2 complex. Thus, in myoglobin, the affinity of myoglobin-O2 is increased, whereas there is no effect of Fe-CO binding in myoglobin. So, the binding affinity between Fe and CO decreases from 20,000-fold in free heme to approximately 40-fold in heme embedded in myoglobin. So, this O2 affinity in globins is physiologically important and thwarts poisoning by carbon monoxide.
The molecular motions or ”breathing” in the structure of myoglobin also control the binding affinity of O2 and heme. Inside the folded polypeptide chain, the heme molecule is deeply buried. So, it has less or limited direct ways for oxygen to displace from the surrounding solution to the ligand binding site.
In a rigid protein, O2 could not quickly enter or exit from the heme pocket. However, transient cavities are formed in the protein structure by the rapid molecular flexing of the amino acid side chains. Due to the formation of these cavities, O2 can make its way through them.
In myoglobin, distal His amino acid controls access to one major pocket located near the heme. It rotates to open or close that pocket within a nanosecond time scale. The distal His function varies among different globins.
Coordination of histidine with heme in other globins
In neuroglobin, cytoglobin, and some other globins of plants and invertebrates, the distal His makes straight coordination with the heme iron at the ligands binding location. In this binding process, the ligand and O2 must have to displace the distal His. After this binding, a hydrogen bond is again formed between O2 and distal His.
Functional comparison between hemoglobin and myoglobin
In animals, all the oxygenated blood is transported by hemoglobin in erythrocytes or red blood cells. Human erythrocytes are formed from hemocytoblasts (precursor stem cells). They range in size from 6 to 9 nm in diameter and appear as biconcave discs.
During maturation, the daughter cells from the stem cells produce large amounts of hemoglobin and deprive their organelles, e.g., mitochondria, nucleus, and endoplasmic reticulum. So, erythrocytes are vestigial cells that are unable to reproduce and live for only 120 days in humans. Erythrocytes mainly carry hemoglobin, dissolved in the cytosol at a high concentration.
Arterial blood passes from the lungs to the peripheral tissues through the heart and consists of hemoglobin saturated with 96% oxygen. The venous blood consists of hemoglobin saturated with only 64% oxygen. Therefore, each 100 ml of blood passing through a tissue releases nearly 1/3rd of the total oxygen it carries.
Hemoglobin is a roughly spherical, tetrameric protein with four heme prosthetic groups. The change in each polypeptide is associated with one heme prosthetic group. The structure of hemoglobin and myoglobin are quite similar, though the amino acid sequences of the three polypeptides are identical at only 27 positions.
Myoglobin functions as a good oxygen storage protein due to its insensitivity to small changes in the concentration of dissolved oxygen. Hemoglobin is a better transport protein than myoglobin due to its multiple subunits and oxygen-binding sites. The subunits of hemoglobin interact among themselves to cause conformational changes in hemoglobin. This change in conformation changes the affinity of the protein for oxygen.
Hemoglobin undergoes structural changes after binding with oxygen
Hemoglobin has two major conformations, the tension state (T state) and the relaxed state (R state). Oxygen can bind with hemoglobin in any of the two states. However, the binding of oxygen with the R state of hemoglobin brings it to a stabilized state. This is because oxygen has a higher affinity for the R state than the T state. The T state is more stable in the absence of oxygen and is the predominant conformation of deoxyhemoglobin.
The T-state is stabilized by more ion pairs lying at the α1β2 and α2β1 interface. When oxygen binds to hemoglobin in the T-state, it leads to a change in conformation to the R-state. During this transition of the protein, the individual subunits undergo a little change. The αβ subunit pairs rotate, minimizing the pocket between the β subunits.
Some ion pairs stabilizing the T-state are broken, and some new ion pairs are formed during this process. According to Max Perutz, when the key amino acid side chains surrounding the heme change their positions, it spurs the T→ R state transition.
Cooperative binding of hemoglobin with oxygen
In the lungs, hemoglobin binds efficiently with oxygen, having a partial pressure of about 13.3 kPa. The oxygen is released into the tissues, having a partial pressure of about 4kPa. In the lungs, a protein binds with O2 with a high affinity and efficiency. However, it wouldn’t release much oxygen in the tissues. A protein binds oxygen with a very low affinity to release it into the tissues and therefore, it will not take up much oxygen in the lungs.
Hemoglobin undergoes a transition from a state of low affinity to a state of high affinity as it binds with more and more O2 molecules. It has a sigmoid binding curve for oxygen (Figure below).
The affinity between hemoglobin and oxygen
The affinity of oxygen in adjacent subunits of hemoglobin can be altered, when O2 binds with individual subunits of hemoglobin. Deoxyhemoglobin weakly binds with the first O2 molecule because the O2 binds to a subunit, the T state of hemoglobin. This binding leads to conformational changes in adjacent subunits, which help in the binding of additional O2 molecules.
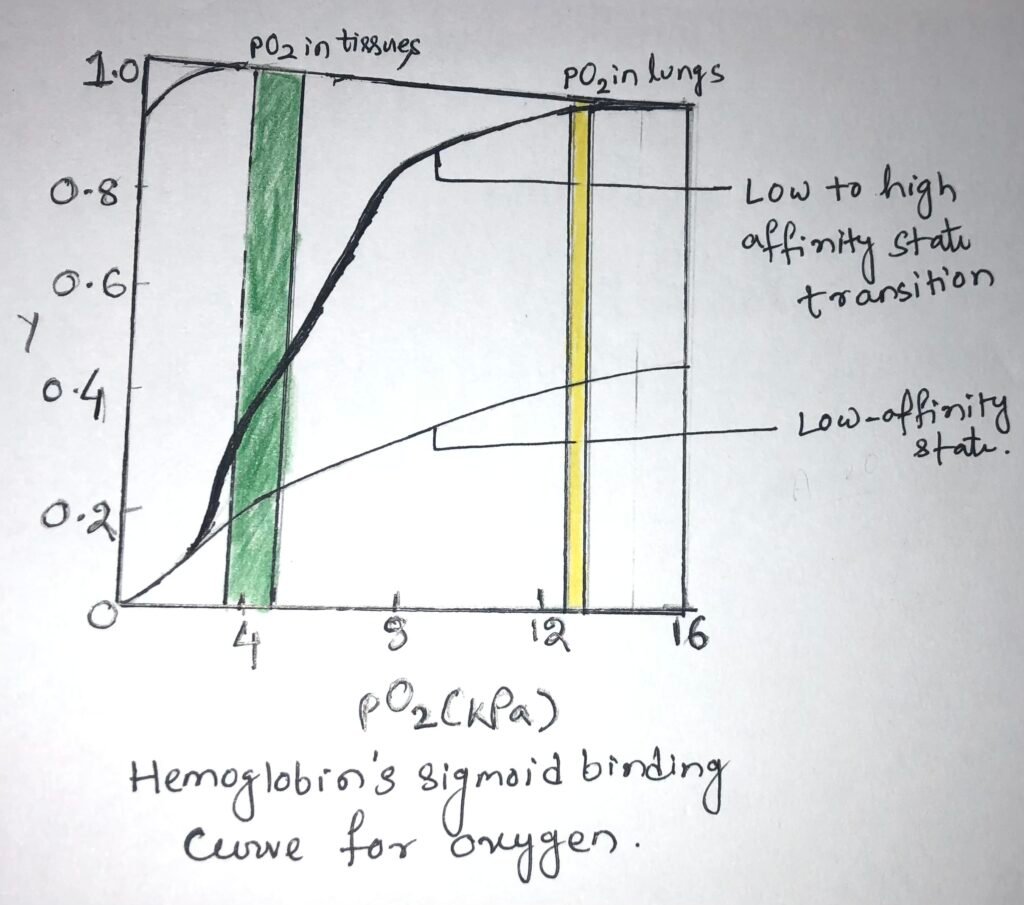
Thus, there is a T state to R state transition that happens quickly in the first subunit. The fourth O2 molecule, which is the last one binds to heme, with an R state subunit. This binding has a much higher affinity when compared to the first molecule.
The MWC and the sequential model
Cooperative binding of oxygen to hemoglobin was first discovered by Archibald Hill in 1910. Structural stability is not uniform throughout a protein molecule. Two models have been proposed for cooperative binding. The first model was proposed by Jacques Monod, Jeffries Wyman, and Jean-Pierre Changeux in 1965, also known as the MWC model or the concerted model.
According to the concerted model, the subunits of a cooperatively binding protein are functionally identical. The two conformations exist for each subunit, and all subunits undergo transition from one conformation to the other simultaneously.
The two conformations maintain equilibrium among themselves. The ligand has the flexibility to bind to both conformations but has more affection towards the R state. According to this model, a protein has no individual subunits in different conformations.
The sequential model is proposed by Daniel Koshland and colleagues. According to this model, the conformational change induced by the binding of a ligand to a protein in one subunit creates a similar change in an adjacent subunit. Thus, it makes a place for the second ligand to bind. This model entails more potential intermediate states than the concerted model.
Hemoglobin is allosteric in nature
When a ligand binding to a protein in one site affects the binding properties of another site of the same protein, the protein is said to be an allosteric protein. The binding of ligands induces the protein to take different shapes or conformations. Thus, ligands act as modulators that interconvert more active and less active forms of the protein.
The modulators may be either inhibitors or activators for the allosteric proteins. If the ligand is a modulator, then the interaction between protein and ligand is homotropic. If the modulator is different than the normal ligand, then the interaction is a heterotropic one.
Proteins having two or more modulators can have homotropic and heterotropic interactions. proteins having two or more modulators can have both homotropic and heterotropic interactions.
The binding of oxygen to hemoglobin is a form of allosteric binding. Oxygen is both considered as a ligand and an activating homotropic modulator. On each subunit, there is only one binding site for O2. So, the allosteric effects produced by co-operativity are mediated by conformational changes transmitted from one subunit to another by interaction between subunits. A co-operative binding shows a sigmoid binding curve.
The allosteric protein in its binding site possesses stable segments in proximity to relatively unstable segments. Unstable segments can cause frequent changes in conformation. The moving parts of the protein’s binding site are stabilized in a particular conformation after binding with the ligand. This affects the conformation of adjacent polypeptide subunits.
Transportation of H+ and CO2 by hemoglobin
Hemoglobin carries nearly all the oxygen required by cells from the lungs to the tissues. It also carries H+ and CO2–, the two end products of cellular respiration, from the tissues to the lungs and kidneys, where they get excreted.
Oxidation of organic fuels in mitochondria produces CO2, which is hydrated to form bicarbonate. This is catalyzed by the enzyme carbonic anhydrase, which is particularly abundant in erythrocytes. If carbon dioxide were not converted to bicarbonate, then it would have resulted in the production of bubbles in the tissues and blood.
The binding of oxygen and hemoglobin is greatly influenced by pH and concentration of CO2. Thus, the interconversion of CO2 and bicarbonate is vital. Hemoglobin transports about 15% to 20% of the CO2 and about 40% of the total H+ formed in the tissues to the lungs and kidneys.
At relatively low pH and high concentration of peripheral tissues, the affinity of hemoglobin for oxygen decreases as H+ and CO2 are bound, releasing oxygen to the tissues.
However, in the lung capillaries, CO2 is excreted, and the pH of blood consequently rises. The affinity of hemoglobin for oxygen also increases. This leads to binding more oxygen for transport to the peripheral tissues.
The effect of pH and CO2 concentration on binding and releasing oxygen by hemoglobin is known as the Bohr effect. This is established by the Danish physiologist Christian Bohr in the year 1904.
Conclusion
Myoglobin and hemoglobin are the two important globin proteins that store and transport oxygen. Myoglobin functions as a good oxygen storage protein, whereas hemoglobin functions as a better oxygen transport protein. It has multiple subunits and oxygen-binding sites, which make it a better oxygen transporter.
Hemoglobin, after binding with oxygen, undergoes structural changes. Hemoglobin undergoes cooperative binding with oxygen. It has a sigmoid binding curve for oxygen. Hemoglobin is an allosteric protein, and binding of oxygen to hemoglobin is a form of allosteric binding.
The binding of oxygen and hemoglobin is highly impacted by pH and concentration of carbon dioxide. Hemoglobin transports about 15% to 20% of the CO2 and about 40% of the total H+ formed in the tissues to the lungs and kidneys.
You may also like:
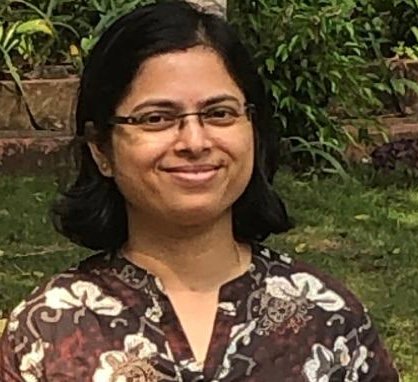
I, Swagatika Sahu (author of this website), have done my master’s in Biotechnology. I have around twelve years of experience in writing and believe that writing is a great way to share knowledge. I hope the articles on the website will help users in enhancing their intellect in Biotechnology.