In this article, I briefly describe the role of G protein-coupled receptors in vision.
G protein-coupled receptors (GPCRs)
These receptors act through a guanosine nucleotide-binding protein or G protein, family member. Signal transduction is defined through three essential components. The three essential components can be described as a plasma membrane receptor with seven transmembrane helical segments, a G-protein that cycles between active and inactive forms, and an effector enzyme in the plasma membrane. A hormone, growth factor, or neurotransmitter acts as the first messenger that activates a receptor from outside the cell. The activated receptor causes its associated G-protein to exchange its bound GDP for a GTP from the cytosol. This causes the dissociation of the G-protein from the activated receptor and binding to the nearby effector enzyme, thus altering its activity.
The human genome encodes over 800 GPCRs. Out of these, about 350 detect hormones, growth factors, and other endogenous ligands. Nearly 500 receptors serve as olfactory and gustatory receptors. GPCRs have been implicated in many human conditions, including allergies, depression, blindness, diabetes, and various cardiovascular disorders. Around 20% of all cancers involve GPCR mutations. More than one-third of the drugs in the market target one GPCR or another.
GPCRs play an important role in vision
In animals, specialized sensory neurons use signal transduction mechanisms similar to those detecting hormones, neurotransmitters, and growth factors. These mechanisms of neurons help in the detection of light, odors, and tastes in animals. Gated ion channels and intracellular second messengers amplify an initial sensory signal. Thus, the system adapts to continued stimulation by changing its sensitivity to stimulus. Integration of sensory input from several receptors happens before the final signal reaches the brain.
Use of classic GPCR mechanism in vertebrate eye
Rhodopsin, a GPCR in the disk membranes of rod cells of the vertebrate eye, is responsible for initiating visual transduction. When light falls on rhodopsin, visual transduction begins. The protein component of rhodopsin is opsin, which is covalently attached to the light-absorbing pigment 11-cis-retinal. Opsin lies near the middle of the disk membrane bilayer.
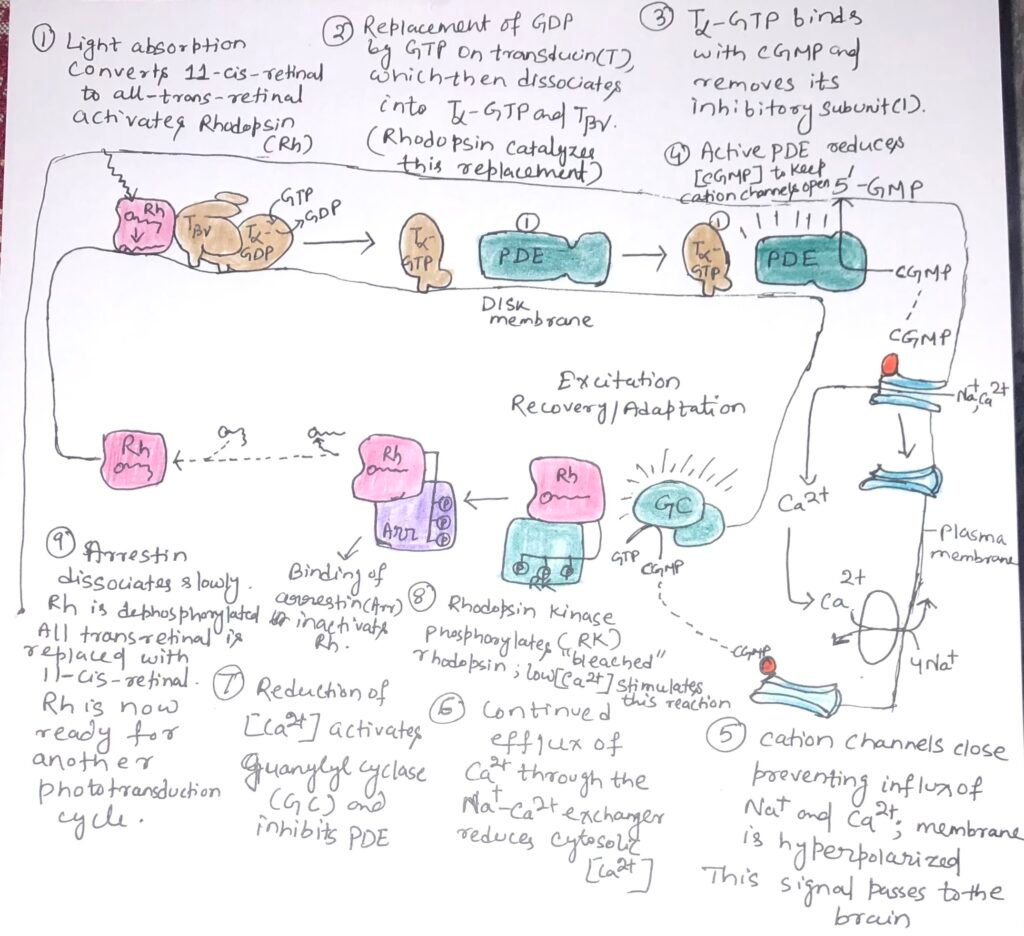
When the retinal component of rhodopsin absorbs a photon, the energy causes a photochemical change and 11-cis-retinal is converted to all-trans-retinal {Figure 1 (1)}. The change in the structure of the chromophore leads to conformational changes in the rhodopsin molecule. Thus, rhodopsin interacts with its trimeric G protein transducin and activates it. Then rhodopsin stimulates the exchange of bound GDP on transducin for GTP from the cytosol {Figure 1 (2)}. The activated transducin removes an inhibitory subunit from the membrane protein cyclic GMP phosphodiesterase (PDE), thus stimulating it {figure 1 (3)}. The activated cGMP PDE degrades the second messenger 3’,5’-cGMP to 5’-GMP, and lowers the concentration of cGMP {figure 1(4)}.
A cGMP-dependent Na+ or Ca2+ channel in the plasma membrane closes {figure 1(5)}, while a Na+ Ca2+ active antiporter continues to pump Ca2+ outward across the plasma membrane {figure 1(6)}. Thus, this makes the transmembrane electrical potential more negative inside. This electrical change passes to the visual cortex of the brain through a series of specialized nerve cells.
Visual transduction is a multi-step process
The process of visual transduction includes many steps that result in a huge signal amplification. Each excited rhodopsin activates at least 500 molecules of transducin. A transducin molecule can activate a molecule of cGMP PDE. Each activated phosphodiesterase can hydrolyze 4200 molecules of cGMP per second. The binding of cGMP to cGMP-gated ion channels is cooperative. Absorption of a single photon closes more than 1000 ion channels, hyperpolarizing the cell’s membrane potential by about 1 mV.
As our eyes read a line, the retinal images of earlier words fade quickly as we perceive the next set of words. During this brief moment, significant biochemical processes occur. Once the rod or cone cells stop being illuminated, the photosensory system promptly deactivates. The α subunit of transducin exhibits intrinsic GTPase activity, leading to the hydrolysis of GTP within milliseconds after the reduction in light intensity. This allows the Tα subunit to rejoin with the Tβγ subunits. The inhibitory subunit of PDE, which had been interacting with Tα-GTP, is released and binds back to PDE, substantially reducing its activity and thereby slowing the breakdown of cGMP.
A second factor that helps to end the response to light is the reduction of intracellular [Ca2+], which is the result of continued Ca2+ efflux through the Na+– Ca2+ exchanger. High [Ca2+] inhibits the enzyme guanylyl cyclase that makes cGMP. Thus, when [Ca2+] falls, cGMP production rises {figure 1(7)}.
Rhodopsin changes its conformation on absorbing light
Rhodopsin on prolonged illumination, undergoes changes that limit the duration of its signaling activity. When rhodopsin absorbs light, its conformation gets changed and exposes many Thr and Ser residues in its carboxy-terminal domain. These residues are phosphorylated by rhodopsin kinase {figure 1(8)}. The protein arrestin 1 attaches to the phosphorylated carboxyl-terminal domain of rhodopsin and prevents further interaction between activated rhodopsin and transducin.
Color vision
Color vision involves a path of the same sensory transduction in cone cells and rod cells. However, in cone cells, it is triggered by a bit of different light receptors. Three different types of cone cells especially detect light from different regions of the spectrum, using three related opsins. Each cone cell expresses only a single type opsin. Each opsin type is closely related to rhodopsin in size, amino acid sequence, and presumably three-dimensional structure. However, the differences among opsins are great enough to place the chromophore, 11-cis-retinal in three slightly different environments. As a result, the three photoreceptors have different absorption spectra (figure 2). By integrating the output from the three types of cone cells, human beings can discriminate colors and hues. Each type of cone cell contains one of the three types of photoreceptors.
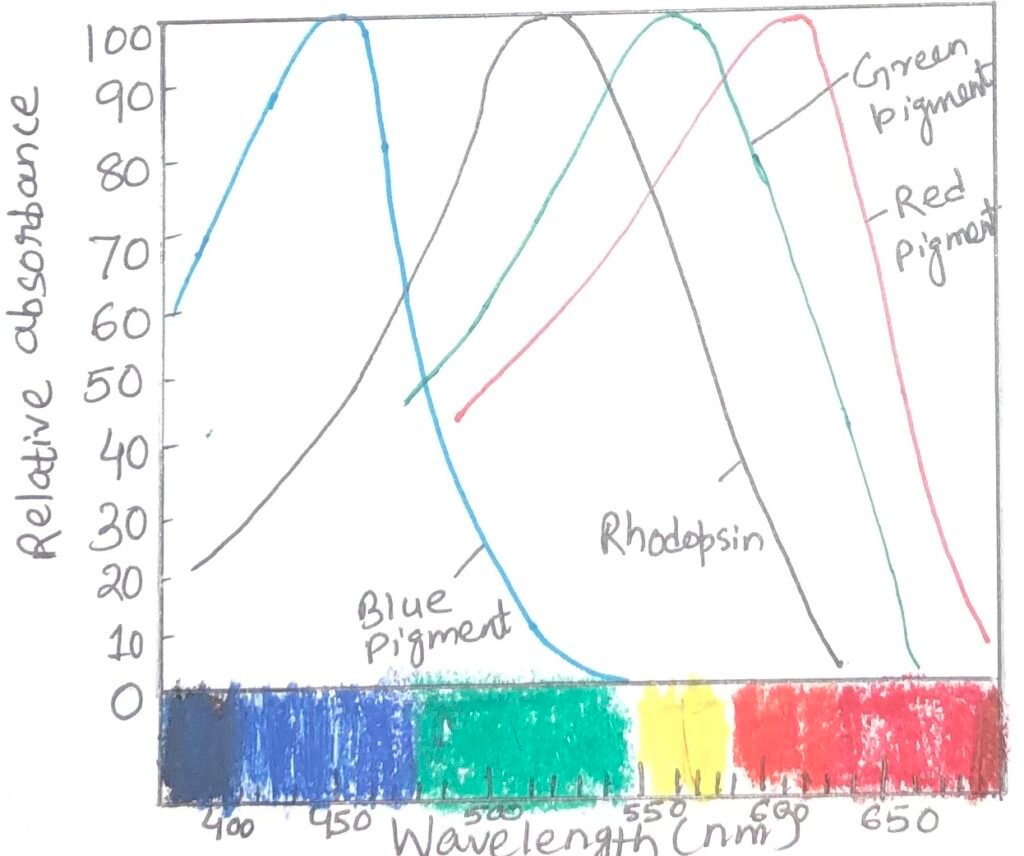
Color Blindness
The inability to distinguish red color from green is known as color blindness. It is a common, genetically inherited trait in humans. The different types of color blindness result from different opsin mutations. One form of the disease is due to the lack of the red photoreceptor. In this case, the affected individuals are red – dichromats. Another form includes the affected individuals as green – dichromats, as they lack green photoreceptors. In some cases, both red and green photoreceptors are present with a changed amino acid sequence that causes a change in their absorption spectra, resulting in abnormal color vision. These affected individuals are red-anomalous trichromate or green-anomalous trichromate depending upon the pigment alteration.
Conclusion
G-protein coupled receptors act through a guanosine nucleotide-binding protein or G-protein family member. Signal transduction is defined through three essential components. The human genome encodes over 800 GPCRs. Out of 800 GPCRs, about 350 detect hormones, growth factors, and other endogenous ligands. Nearly 500 receptors serve as olfactory and gustatory receptors. GPCRs have been embroiled in many human conditions like allergies, depression, blindness, diabetes, and various cardiovascular disorders.
Rhodopsin, a GPCR in the disk membranes of rod cells of the vertebrate eye is responsible for the initiation of visual transduction. When light falls on rhodopsin, visual transduction begins. The protein component of rhodopsin is opsin, which is covalently attached to the light-absorbing pigment 11-cis-retinal. The process of visual transduction includes many steps that result in a huge signal amplification.
As our eyes read a line, the retinal images of earlier words fade quickly as we perceive the next set of words. During this brief moment, significant biochemical processes occur. Once the rod or cone cells stop being illuminated, the photosensory system promptly deactivates. Color vision involves a path of the same sensory transduction in cone cells and rod cells. The inability to distinguish red color from green is known as color blindness. It is a common, genetically inherited trait in humans. The different types of color blindness result from different opsin mutations.
You may also like:
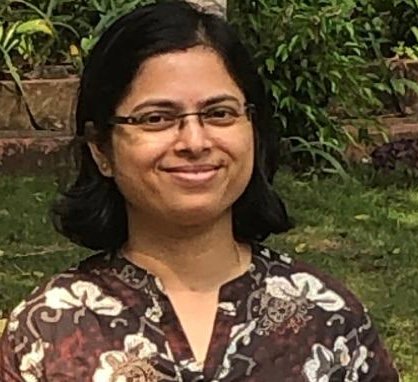
I, Swagatika Sahu (author of this website), have done my master’s in Biotechnology. I have around twelve years of experience in writing and believe that writing is a great way to share knowledge. I hope the articles on the website will help users in enhancing their intellect in Biotechnology.